 |
Dislocations are characterized by |
|
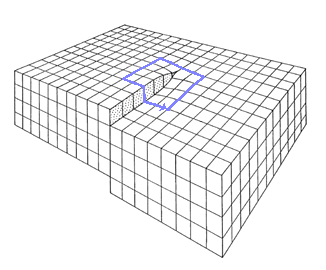 | Burgers circuit for a screw dislocation |
|
 |
1. Their Burgers vector b= |
|
|
|
- vector describing the step obtained after a dislocation passed through the crystal.
- Vector obtained by a Burgers circuit around a dislocation.
- Translation vector in the Volterra procedure.
|
|
|
 |
All definitions of b give identical results for a given
dislocations; but watch out for sign conventions! | |
|
 |
By definition, b is always a translation vector T
of the lattice. | |
|
 |
For energetic reasons b is usually the shortest translation vector of
the lattice; e.g. b=a/2 <110> for the fcc
lattice. | |
 |
2. Their line vector t(x,y,z)
describing the direction of the dislocation line in the lattice |
|
|
 |
t(x,y,z) is an arbitrary (unit) vector in principle
but often a prominent lattice direction in reality | |
|
 |
While the dislocation can be curved in any way, it tends to be straight (=shortest possible
distance) for energetic reasons. | |
| | |
| |
 |
The glide plane by necessity must contain t(x,y,z)
and b and is thus defined by the two vectors |
|
- a=90 o: Edge dislocation.
- a=0 o: Screw dislocation.
- a=60 o: "Sixty degree" dislocation.
- a=arbitrary : "Mixed" dislocation.
|
|
|
 |
The angle a between t(x,y,z)
and b determines the character or kind of dislocation: |
|
|
 |
Note that any plane containing t is a glide plane for a screw dislocation. |
|
|
| |
| |
 |
Dislocations have a large line energy Edis per length
and therefore are never thermal equilibrium defects | |
|
| |
| |
|
 |
The formal Volterra definition of dislocations is very useful and extendable to
more complex kinds of dislocations | |
|
|
 |
Cut into the lattice with a fictitious "Volterra"
knife (make a plane cut to keep it easy), The cut line is a closed loop by necessity. The part of the cut line inside the
crystal identifies the dislocation line. | |
|
 |
Move the part of the lattice above (or below - attention,
signs change!!) the cut plane by an arbitrary lattice translation vector=Burgers vector of the dislocation. Add or remove
lattice points as necessary (=remove or fill in atoms in the crystal
going with the lattice). | |
|
 |
Mend the lattice (or crystal)
by "welding the upper part to the lower one. There will be a perfect fit by definition everywhere except along the
dislocation line. | |
|
 |
Make the best arrangement of the atoms along the dislocation line by minimizing their energy
(make best possible bonds). | |
 |
You now have formed a dislocation. |
|
|
 |
The procedure is "easily" extended to dislocations in n-dim. lattices,
to (special) dislocations with a Burgers vector not defined as translation vector of the lattice and to lattices more complex
than a simple crystal lattice. | |
| |
| |
 |
Direct consequences are: |
|
|
|
 |
A dislocation cannot just end in the interior of a crystal |
|
|
 |
There is a "knot rule" for dislocation knots:
Sb=0 provided the signs of the line vectors follow a convention (all pointing to or
away from the knot) | |
|
 |
There can be all kinds of dislocation loops (just confine
your fictitious cut to the lattice interior!) | |
| |
| |
 |
Note: "Simple" geometric considerations allow to deduce a lot
about properties of dislocations | |
|
© H. Föll (Defects - Script)